Black holes throttle star formation
The brighter the black hole shines, the fewer stars from nearby.
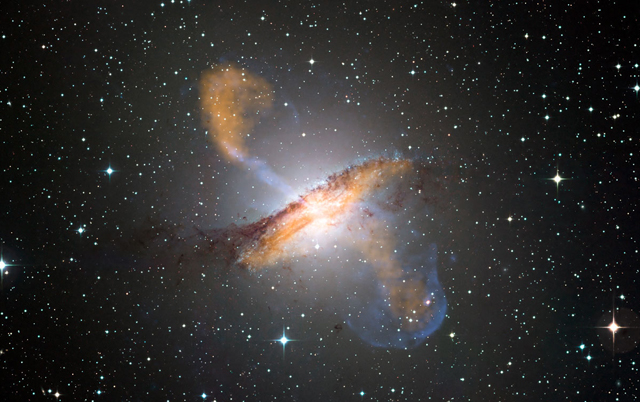
An active galactic nucleus, powered by a black hole.
What sets the limits on a galaxy's size? It might be the supermassive black hole sitting in its core. Many observations indicate the mass of the central black hole in a galaxy is correlated with the mass of the central region of the galaxy itself.
A widely accepted model states that galaxies and the supermassive black hole share an evolutionary relationship. During the early days, when a galaxy is young, the raw ingredients for both star formation and black hole growth are common. However, as the black hole becomes active through consuming gas, the matter it's drawing in blasts the star-forming medium away through powerful winds, slowing the birth rates of new stars.
While this model is clear, direct evidence for it has been lacking: the black holes drive processes that shine very brightly, dominating the light coming from star formation in most wavelengths. Nevertheless, researchers used the Chandra Deep Field North (CDF-N) X-ray survey and the submillimeter instrument on the Herschel Space Observatory to measure both the star formation rate and black hole activity. As M. J. Page et al. report in Nature, they found rates of star birth in galaxies slowed down once the central black hole became active. This result provides strong support for the shared evolution of galaxies and the black holes at their hearts.
The researchers targeted 176 galaxies with active galactic nuclei (AGN)—supermassive black holes surrounded by matter that pumps out huge amounts of energy in the form of light—from the CDF-N survey. Within that sample, they looked specifically at the 65 galaxies that formed 2 to 6 billion years after the Big Bang, when star formation and black hole growth were strongest.
During active periods, the supermassive black hole in the nucleus of a galaxy is extremely luminous. These AGN shine in a wide range of wavelengths—including X-rays—as they gorge themselves on a diet of gas. Some of the gas falls in or orbits the black hole in the form of accretion discs, emitting light during the high acceleration; other gas is blasted out in jets. AGN ramp up their intensity gradually, based on the available fuel.
To assess star formation, the researchers examined the galaxies using 250 micron light (0.25 millimeters), which is a wavelength not associated with AGN activity—but is produced strongly by newborn stars. In addition, 250 micron light punches through the dusty environment in which stars form, so it's ideal for studying such systems. On the other hand, submillimeter observing must be done from orbit, since these wavelengths don't penetrate Earth's atmosphere. This obstacle has prevented the test of the coevolution model until now; that's why the Herschel observations were needed.
Within the sample of 65 galaxies formed early in the Universe's history, Page et al. divided the AGN into two categories based on the brightness of their X-ray emission, which should relate to the black hole's activity. In the lower-luminosity category, they found 44 AGN, with 11 having strong 250 micron emissions, indicating star-formation. In the higher-luminosity category, they found no galaxies with star formation signatures out of 21 AGN. Based on this data and a statistical model, they estimate 25 percent of all lower-luminosity AGN should be undergoing star formation, while less than 5 percent of higher-luminosity AGN do. The errors on both of these estimates are fairly large, partly due to the relatively small sample size.
Using 350 and 500 micron light along with the 250 micron observations, the researchers estimated the star formation rate in each galaxy. Plotting this against the X-ray luminosity for the AGN, they found a suggestive relationship: for lower luminosities, more activity in the black hole was associated with increased star formation. But, when the AGN reached a critical level, star formation decreased steeply with AGN luminosity. This suggests that the black hole winds may be depleting the galaxy of usable material for star birth. Furthermore, in the sample that includes galaxies closer to the Milky Way, Page et al. found decreased star formation for all ranges of AGN, indicating that the available fuel both for star formation and for driving black hole activity had diminished.
Since star formation peaks before AGN luminosity, that's a strong indicator that black hole activity suppresses star formation, as Page et al. point out. This data indicates that the black hole/galaxy coevolution model is correct, and additional observations should settle the issue by reducing the statistical uncertainties.
Black holes? They’re all the same
Properties of their jets scale from solar masses to supermassive.
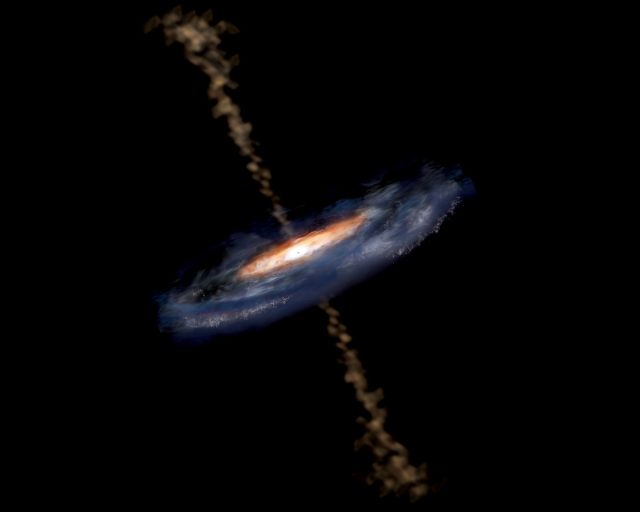
An artist's conception of the jets produced by an active galaxy.
Recently, we discussed the discovery of a black hole in a neighboring galaxy. It appears to be swallowing matter at a rate that's close to the theoretical limit on this process. This discovery raises the hope that we'll be able to get a clear view of the environment around the black hole, where the inflow of matter leads to the formation of particle jets that expel some of the matter at relativistic speeds. This process is also thought to power quasars, where the jets of supermassive black holes generates some of the brightest objects in the Universe.
But there's a small caveat that should temper astronomers' enthusiasm: we don't know for a fact the same process that powers jets from small black holes can scale up to objects that may weigh billions of times the mass of the Sun. Conveniently, in the same week this discovery was announced, another team of astronomers has provided evidence that all of these jets have similar properties.
Although black holes are happy to swallow most of the matter that comes their way, a bit of it escapes—if by "escapes" we mean "gets shot away from the black hole at nearly the speed of light." These jets of particles are so energetic that, in at least some cases, they're entirely thrown out of the galaxy where the black hole resides. Various models indicate the black hole's intense magnetic field lines power the jets by latching on to charged particles in the matter that is falling in towards the event horizon.
We've got some great models of how this can work and how the environment narrows and focuses the jets, sending them in a beam lined up with the black hole's north and south pole. But we're only just starting to resolve some of the features near the black holes at the center of quasars. Given that most quasars are quite distant and the core of galaxies are crowded places, there are likely to be limits to how well we can ever resolve the details of the environment that forms the jets.
That explains the appeal of objects like gamma ray bursts (which result from a black hole's formation) and microquasars (stellar-mass black holes actively swallowing matter). These have been found much closer to Earth (including in our own galaxy) and don't necessarily reside in the galactic center, which means we should be able to get a relatively unobstructed view of one. And, with a bit of luck, we could potentially get a clearer perspective on the jets' formation.
But that raises the question of whether what we learn from these smaller bodies is actually relevant to black holes. Remember, these are so big that, if you dropped one in place of the Sun, its edges would extend out past the most distant planets. Earlier studies had suggested that microquasars and the full-sized versions operate through a similar process. The new study now extends this to gamma-ray bursts.
The authors searched for any reports of observations of the jets of black holes, ranging in size from the gamma-ray bursts that accompany the formation of black holes up to the blazars that are generated by supermassive black holes in galactic cores. These objects create gamma ray emissions as charged particles are whipped around at the base of the jets as they form. They also used radio observations to track the total energy in the jets.
When the two values were plotted against each other, the resulting points all fell along a single line. This suggests there's a simple, linear relationship between the two: the more energy in the jets, the stronger the gamma rays they can produce. So perhaps, there is indeed a single mechanism for accelerating the particles into a jet, one that works across all masses of black holes. The apparent mass independence should help constrain the models we make of jet formation and will mean that any information we get from one object will tell us about the remaining ones.
The curious case of the hidden black hole
Newly described system explains why we've seen fewer black holes than expected.
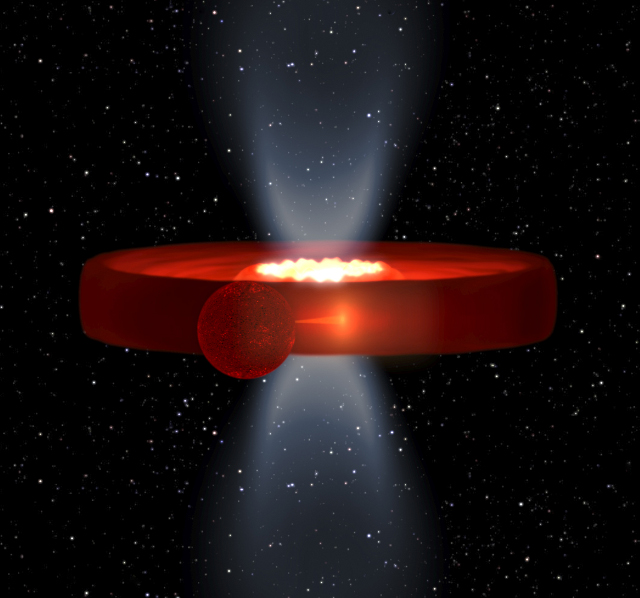
Simulation of a black hole with a companion star and thick, donut-shaped accretion disk. The disk obscures the black hole from our view, which may help explain why we see fewer of these systems than expected.
If you want your pet black hole to be visible, you must feed it regularly. Only when a black hole gorges on a steady diet of gas or other matter does it shine. The disk of matter that orbits it heats up and emits large amounts of light, especially in X-rays. If you have one of the supermassive black holes at the centers of galaxies, feeding it matter can create one of the brightest objects in the Universe.
But the smaller ones should also be pretty visible. And while astronomers expect and have observed black holes comparable in mass to stars, their numbers are fewer than expected, even after decades of searching. Perhaps, as a new paper suggests, this is because many black holes are hidden by an opaque, donut-shaped disk of matter.
J. M. Corral-Santana and colleagues based this hypothesis on a detailed study of a relatively faint, fluctuating X-ray source in the Milky Way. Their observations in X-ray and visible light revealed the signs of a binary system: an ordinary star in orbit around a black hole, similar to other systems, but with some key differences. For one, the star and black hole were so close together that the orbital period of the system was only 2.8 hours. For another, the matter being drawn off the star was obscuring the black hole when viewed from Earth. The authors hypothesized that many other black holes may be similarly hidden, and future searches should take that possibility into account.
Stellar-mass black holes are the remnants of the cores of stars which exploded in supernovae and are at least 20 times the mass of the Sun. Black holes in this mass range have been discovered in binary systems, where their companion is an ordinary star. The transfer of mass from the companion onto the black hole creates an accretion disk: a hot, rapidly rotating platter that emits a great deal of X-ray light. The first black hole discovered, Cygnus X-1, was found through these emissions.
However, in nearly 50 years of X-ray observations, only about 50 black holes candidates have been known in the Milky Way, of which only 18 are confirmed. None of them exhibit eclipses, where the companion star or accretion disk block the X-ray emission. That's a somewhat surprising result, as it may imply we're only identifying systems we see from a privileged angle, one where our telescopes peer "down" onto the system. Since a bright black hole system that undergoes eclipses was identified in the M33 (Triangulum) galaxy, astronomers know it does happen.
But if the orientation of the binaries was random, we would expect about 1/5 of all systems to exhibit strong eclipses. We've seen nothing like that number, so obviously something is amiss.
The current paper examined a faint X-ray source, Swift J1357.2-093313, discovered by the orbiting Swift observatory in 2011. This object was not obviously a black hole from its X-ray signal, but the authors followed up in visible light using the Isaac Newton and William Herschel Telescopes. They found the telltale signs of an accretion disk: two strong peaks of light representing the portions of the disk moving toward us and away from us. (This is due to the Doppler effect: light emitted from material moving toward the telescope will have a shorter wavelength, while material moving away will have longer wavelength.)
Studying the orbital properties of the system, the researchers concluded that the disk rotates around the binary system's shared center of mass once every 2.8 hours, indicating the star's partner is an object about 3 times the mass of the Sun. That relatively large mass rules out other candidates like white dwarfs or neutron stars. The astronomers also identified strong regular variations in the accretion disk's visible light output, with no corresponding X-ray variation. That indicated ripples in the disk, with matter moving inward toward the black hole.
Together, this data strongly indicated a thick, donut-shaped accretion disk that hides the black hole by keeping most of its X-ray emissions from reaching our telescopes. Since the X-ray signature was so weak, the system could only be seen because it is relatively close to Earth. That means astronomers should pay greater attention to other weak X-ray emitters. But it also indicates that many black holes may be too faint to see or may be obscured by other gas in the galaxy.
Nevertheless, the implication is clear: if other black holes are obscured by their accretion disks, it would explain why astronomers have failed to find more stellar-mass black holes in the galaxy, and it could give us a better idea of how many are truly out there.
Neutron stars and black holes illuminate dark matter
Neutron stars' failure to collapse into black holes limit dark matter.
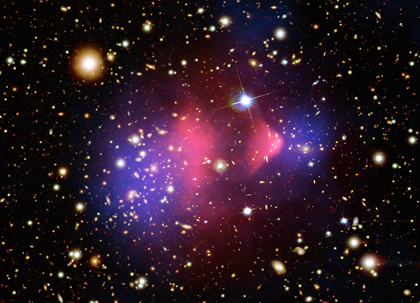
A Bullet Cluster
It is early days yet, but astrophysicists and high energy physicists are starting to get a little nervous. We know from astrophysical and cosmological observations that much of the matter in the universe is invisible to us. Indeed, the bullet cluster—a must to mention in every article written about dark matter—provides spectacular supporting evidence for dark matter. But these observations don't do a lot to pin down the properties of dark matter, other than that it is... dark: it doesn't play with electromagnetic forces (light and charge) very often.
This was all fine before the Large Hadron Collider switched on. The LHC was expected to turn up a slew of new particles—particles required to complete the standard model of physics—some of which might have the right properties to be dark matter. Unfortunately, these particles are yet to appear. They could still appear, rising out of the muddy data like a hungry crocodile. Until then, however, astrophysicists have to use the rest of the Universe as their observatory to pin down dark matter. Neutron stars provide a perfect natural experiment, because, their failure to collapse into black holes tells us a lot about how fast dark matter aggregates.
Although this sounds like something very complicated, it is nothing more than measuring friction. Consider a pendulum: it swings back and forth through its rest position. As time goes on, the oscillations get smaller and smaller, until, finally, it comes to rest again. But, if there was no friction—friction in the joint of the swing, or drag from the air about the arm and mass, etc—then it would swing forever.
This same physics applies to matter in the vicinity of stars. The Earth is in a stable orbit about the sun because the constant acceleration due to gravity is much greater than the frictional slowing that it experiences as it passes through the dilute wind of charged particles that permeate space around a star. But, this is not always the case. A perfect example of this are low Earth orbit satellites. The thin atmosphere of the Earth provides sufficient drag that, eventually, the satellite spirals in and burns up in the Earth's atmosphere.
Moving back to dark matter and neutron stars. A neutron star moves through a galaxy that has a thin, relatively uniform halo of dark matter scattered through it. The massive gravitational field of the neutron star sets the dark matter into motion. Much of the dark matter simply escapes, but some ends up orbiting the neutron star—it should be noted that this probably includes orbital paths that pass through the star. Over time, the density of the dark matter orbiting a neutron star should increase. And, if that dark matter eventually spirals in, the mass and gravitational field will increase until a black holes forms.
Chris Kouvaris, from Denmark, wanted to see if this told us anything about how dark matter interacts with itself. But, to be consistent with everything else we know about dark matter, these collision have to be of a rather special nature. The chance of mutual annihilation must be very small compared to collisions that simply change the speed and direction of the particles (including those that release some energy as light, slowing both particles).
We already know from galactic, and larger, scale measurements that dark matter doesn't play with ordinary matter. These measurements also limit how often dark matter particles collide off each other. But these are all low-density situations, and, if dark matter is restricted to short-range exchange of forces—like the attractive forces that hold the atomic nucleus together—then this would be basically unnoticeable. In other words, the strength of the collisions could be quite strong, but so rare that they just don't matter. In a neutron star environment, what was rare in ordinary space, may become a lot more frequent, so they allow us to test whether collisions between dark matter particles are strong enough to slow them down and allow them to accumulate at the center of a neutron star.
The answer: dark matter doesn't accumulate very fast at all. More specifically, Kouvaris used the expected dark matter density to calculate the possible rates of dark matter accumulation. This accumulation rate depends on the details of the model, so the result is a range of dark matter particle masses, force carrier masses, and collisional frequencies that would result in nearby neutron stars to have collapsed into black holes already. This, along with cosmological observations, and, yes, the bullet cluster observations, result in an exclusion region, for which we can say "dark matter cannot be here."
Although Kouvaris' calculations don't exclude vast swathes of dark matter possibilities, every little bit helps. What I like is that it is an elegant bit of work that combines hard experimental data with speculative models that also give us hints about what to look for if we want to narrow the search for dark matter even further.
Better black hole mass estimates in hours
Watching carbon monoxide swirl around a black hole sizes it up quickly.
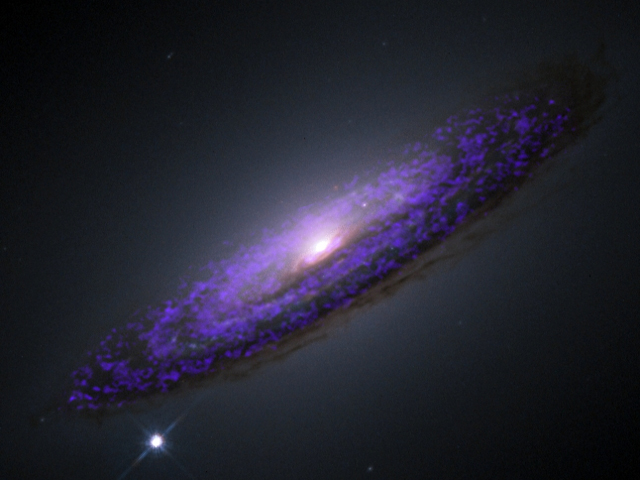
A Hubble Space Telescope image of the galaxy NGC 4526, overlaid with submillimeter observations (shown here in purple). The submillimeter light shows the presence of molecular gas, which could assist in estimating the mass of the galaxy's central black hole.
The data is good: nearly every large galaxy has at least one supermassive black hole in its nucleus. The data is bad: there generally seems to be a connection between the mass of the black hole and the size of the galaxy's bulge, at least for many galaxies. And the data is ugly: accurate direct measurements of black hole masses are hard to come by, since they're buried deep in the middle of their host galaxies.
A promising technique has used gas dynamics in the galaxy as a black hole mass tracer, as proposed by Timothy A. Davis, Martin Bureau, Michele Cappellari, Marc Sarzi, and Leo Blitz. They tested their hypothesis on a galaxy that has a large amount of carbon monoxide emissions, prime for observations with submillimeter observatories. This method requires far less observation time than many other techniques, meaning that many supermassive black holes could potentially be surveyed.
Measuring supermassive black hole (SMBH) masses is not always straightforward. Astronomers can estimate masses by tracking stars in the central regions of galaxies as they orbit the black hole or by mapping emission by nearby ionized gas. Another promising method used X-ray and radio light for the most massive galaxies in the Universe. But these techniques aren't possible for all galaxies
Molecular gas, however, is present in a large number of galaxies, and many molecules emit at wavelengths that can provide high-resolution looks into the regions near SMBHs. These molecules include hydrogen (H2) and carbon monoxide (CO). The latter is particularly interesting, because it emits light in the submillimeter range, the portion of the electromagnetic spectrum lying at the boundary between infrared and microwave light.
Gas near galactic centers moves under a variety of gravitational influence, including the internal gravitational attraction of the gas cloud itself. If the galaxy is host to a SMBH, then it will contribute to the gas motion—its kinematics—as well. The motion of the gas can be measured through the Doppler effect, the shifting of the wavelengths due to the gas' velocity relative to Earth.
The authors of the new study measured CO emission from the galaxy NGC 4526. This is a lenticular (lentil-shaped) galaxy—effectively a spiral galaxy with no spiral arms. Due to its shape, NGC 4526 has a clearly defined central bulge, and that's where the influence of a SMBH would be felt by the molecular gas. The astronomers performed their observations with the Combined Array for Research in Millimetre Astronomy (CARMA), a set of six telescopes in California.
Using multiple telescopes in combination often provides higher resolution than individual telescopes can achieve. That allowed the authors to peer at the central region of NGC 4526 and create a full Doppler map of the CO motion.
From this map, they fit a variety of possible models that could explain the gas kinematics: no SMBH, or a range of possible SMBH mass values. This comparison allowed them to decisively rule out the "no SMBH" option, since that would imply more gas than they actually observed. The best fit to the data included a SMBH roughly 450 million times the mass of the Sun—a hefty black hole, but nowhere close to the biggest known specimen.
As with other gas measurements, the molecular gas observations could run into trouble if the black hole causes turbulence or if the rotation of the gas doesn't align with the stars of the bulge. For NGC 4526, this problem didn't seem to exist, but the authors warn that for other galaxies, it could lead to overestimates of the SMBH mass.
Besides the relative abundance of molecular gas in many galaxies, the method also has a striking advantage: short observation times. With arrays of submillimeter telescopes such as the Atacama Large Millimeter/Submillimeter Array (ALMA) in Chile, the researchers estimated a single black hole could be studied in 5 hours—an impressively short time. That opens up the possibility of performing SMBH surveys, collecting sufficient data to settle questions of black hole-galaxy interaction and coevolution.
A trustworthy guide to black hole astronomy
Gravity's Engines presents the best of modern knowledge of black holes.
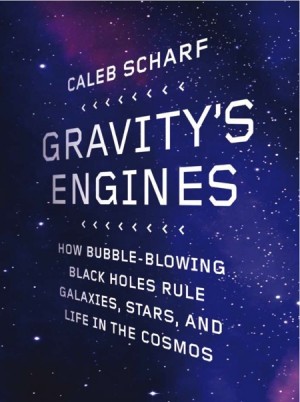
In Caleb Scharf's forthcoming book, Gravity's Engines, the focus is on the observed and observable properties of astrophysical black holes. The subtitle—How Bubble-Blowing Black Holes Rule Galaxies, Stars, and Life in the Cosmos—may be a trifle grandiose, but the book itself is an excellent overview of the state of black hole research. In fact, to explain why black holes are so important, Scharf provides a tour of much of modern astronomy and cosmology along with some requisite history, an impressive feat for such a relatively short book.
After a conceptual introduction to black hole theory, Scharf begins with the fascinating (albeit incorrect) "dark star" model by 18th century mathematicians John Mitchell and Pierre-Simon Laplace. Modern black holes, based on general relativity, are introduced via Karl Schwarzschild's original calculation (performed in the trenches of the Russian front in World War I) and the famous paper by J. Robert Oppenheimer and Hartland Snyder. (Scharf doesn't mention Snyder, though admittedly he says Oppenheimer was "one of those who developed the physics" leading to our modern understanding of gravitational collapse.) Along the way, Scharf describes stellar evolution, white dwarfs, and neutron stars, to show that stellar-mass black holes—as opposed to supermassive black holes (SMBHs) found in the centers of galaxies—arise as the logical continuation of those other stellar remnants.
However, Scharf's main goal is to explore how the biggest black holes evolved with their galaxies, and how this shaped the larger environment. To accomplish that goal, he delves into cosmology: the contents and history of the Universe. Since galaxies and galaxy clusters arise from dark matter structures, and SMBHs help shape both of those things, the understanding of structure formation is a part of the tale. Scharf weaves the disparate strands of this tale together very well, so even though many pages may go by without a single mention of black holes, by the time the main thread appears again, it's clear why the other strands were introduced.
Scharf is very fond of metaphors like the "weaving" one in the paragraph prior. Sometimes those metaphors are very effective: his "cosmic bag" analogy for describing the contents of the Universe in the proper proportions is wonderful. (I'm reminded of Edward Harrison's "cosmic box" metaphor, which I have used on many occasions.) Similarly, Scharf's "map of forever", which includes both space and time to show how intricately linked every aspect of cosmology is, provides a wonderful metaphor.
On other occasions, Scharf lets his metaphors run away with him: when describing how black holes feed back into their environment, he uses a runaway car, a soufflé, and a bowl in the same paragraph as analogies for the same process. While it's probably true that no single metaphor is sufficient for complex physical systems, mixing several together can be distracting. However, this is a minor quibble: for the most part, Scharf uses his analogies effectively, and (in my opinion at least) metaphor is a necessary means to the end of explaining high-level science to a general audience.
After describing how black holes shine brightly and pump particles back out into space, Scharf turns his attention to the work to which he contributed. While his current job as Columbia University's Astrobiology Center mostly deals with exoplanets, his earlier work involved studying SMBHs in early galaxy clusters. Particularly, he brings this forward to argue that certain types of SMBHs in galaxies may make them habitable for life, an interesting and somewhat controversial theory. It's definitely true that central black holes and their host galaxies evolve together in a fascinating and complicated way, so Scharf's idea may turn out to be correct. As always in science, it's about the evidence for the argument, not the elegance behind it, but it's an intriguing idea that will make readers think.
The book assumes no strong prior knowledge of black holes or astrophysics, but by the time it ends, I think readers should have a good idea of the state of the art. While Scharf's approach is not the same as that of Stephen Hawking or Brian Greene, his explanations are complementary to theirs. Anyone left wondering whether black holes are just too exotic to be real from the more quantum approach will find good answers in Gravity's Engines.
No comments:
Post a Comment
Let us know your Thoughts and ideas!
Your comment will be deleted if you
Spam , Adv. Or use of bad language!
Try not to! And thank for visiting and for the comment
Keep visiting and spread and share our post !!
Sharing is a kind way of caring!! Thanks again!