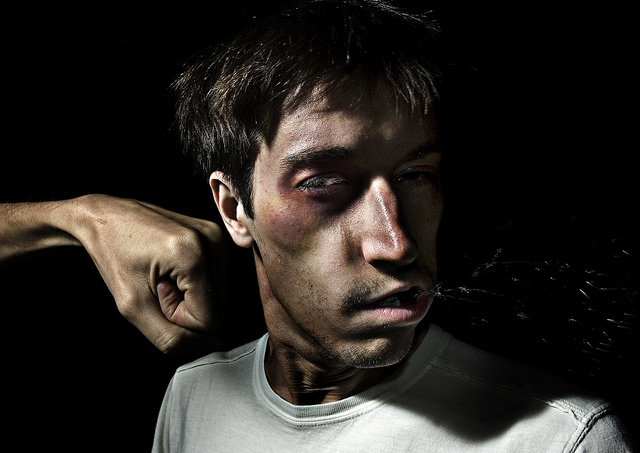
Physicists are measuring atoms by punching them in the face, so to speak.
Those of you who know me know that I get excited by clever tricks. So, sometimes I take a break from writing about the latest and greatest scientific results, and talk about some cool new way of performing an experiment. You can think of these sorts of developments as enablers of better research.
Recently, a group of researchers has shown a very simple way to control light pulses with unheard of precision, which will make a whole class of common physics experiments accessible to many more researchers.
I punched him in the face to see how the crowd would react
Before we get to their cool trick, I want to describe a very common physics experiment, called a pump-probe experiment. Imagine that you want to test and understand the full range of human interaction. You could spend years observing people in different situations, noting their reactions and drawing correlations between behavior and environmental stressors.
A physicist would probably not take this approach. Being simple minded, we would walk into a room full of people, punch someone very hard in the face, and walk out again. We'd then observe all the people in the room, noting their reactions to the initial event, to each other, and to whoever entered the room as a function of time. Essentially, we use one shocking event to probe as large a range of human behavior as possible.
Regretfully, these experiments are unethical, so physicists get their kicks by being cruel to atoms and molecules instead. The same experimental approach is taken: a large and very short laser pulse kicks a bunch of atoms into a highly excited state. We then follow that with a second, weaker laser pulse that measures some aspect of the atoms. For example, the second laser pulse might attempt to kick an electron out of an atom, but can only do that if the first put the electron in a specific state.
To give you an idea of the time scales we care about, electrons do interesting things in about a femtosecond (10-15s), so a short sharp shock needs to be in the attosecond (10-18s) region. Likewise, to measure where or what an electron is doing right now requires a laser pulse with attosecond duration.
Generating these light pulses is relatively simple. A charged particle, like an electron, will radiate light when it is accelerated. To get attosecond pulses, one simply needs to put an electron through a very short, powerful acceleration. But where would you get a large accelerating field? A pulse of laser light, of course. Certain lasers can produce pulses of light that are just a few femtoseconds in duration, but have huge intensities. These fields are so strong that, when they encounter an atom, they simply rip an electron away from it and give it a short, very violent joy ride.
Although the electron is initially driven out of the atom and accelerated away, the electric field associated with light oscillates. So, as the electric field changes sign, it drives the electron back to the atom, where it slams back into place. All the energy gathered by the electron during this process is released during that moment of violent deceleration as a single, high-energy photon.
By carefully controlling the relationship between the laser light pulse envelope—the flash that one would see if you could measure fast enough—and the electric field that makes up that pulse, one can ensure that very short light bursts are emitted. Hence, attosecond pulses are... easy. Simply blast a laser beam through a gas jet.
So, getting the pulses are easy. Using them experimentally is more tricky, because you need to control the timing between two pulses. We ask the electron "What are you doing right now?" But, actually, right now means "in the time since we gave you a good kicking." We know the two laser pulses—one to do the kicking and one to do the asking—have attosecond duration, but to get reliable answers, we need to know the time delay between them to much less than attosecond precision.
Like getting a hair to sit still in Manhattan
But timing is a nightmare. Light travels at about 300 million meters per second, so in an attosecond, a light pulse travels only 0.3nm. Even the movement of mirrors due to thermal effects, passing air currents, and mechanical vibrations will produce changes that are much, much larger than that. So the timing problem comes down to one of mechanical stability: controlling the relative positions of a pair of mirrors to within 0.3nm. That can be done, but only to within 10nm. It involves rather dramatic amounts of technology, dedicated technicians, and a huge budget.
The only alternative is to be really clever.
Remember above I said that you need to control the relationship between the light's underlying electric field and its pulse envelope to generate short pulses? This also controls where within the overall light pulse the attosecond pulse is generated. Under ordinary conditions, the phase of the light (which is related to its electric field) doesn't change: if I measure the phase at one location and then let the light propagate a few meters, I will find no change.
But this is not true when light passes through the focus of a lens. In the region of the focus, the light behaves as if it is travelling slightly faster—indeed the phase of the light is travelling faster. So, the phase relative to the envelope of the pulse changes by a half cycle over a few millimeters. So by placing two sources of atoms (typically, jets of gas) at different locations relative to the focus of the lens, we can create two attosecond pulses with a defined separation.
Let me explain that another way. If I had no lens, but just two gas jets separated by five millimeters, then the light encounters the first gas jet and generates an attosecond pulse. That travels with the light beam to the second gas jet where it generates a second attosecond pulse that overlaps perfectly with the first. You simply get a more intense attosecond pulse. From an outside perspective, you have a single attosecond pulse, and the number of gas jets makes no difference.
Now, we add a lens. The first gas jet is five millimeters before the focus and the other is right in the focus of the lens. The phase shift due to the focusing of a lens, causes the second attosecond pulse to be generated slightly earlier within the laser pulse than expected, so the two attosecond pulses do not overlap. From the perspective of the experimenter, two attosecond pulses are generated, and their separation depends on where the two gas jets are with respect to the focal point of a lens.
Suddenly, instead of worrying about the stability of two mirrors with respect to each other, all you need do is place two gas jets at different locations within a light field.
To put it in perspective, a change of about 15mm in one gas jet position changes the timing between two attosecond pulses by, at most, a femtosecond. That means both that you have very precise control over the timing, and that small changes in the position of the gas jet don't change the delay all that much. Indeed, the researchers showed that their pulse generation scheme was stable to within 100zs (10-21s). To do this using normal optics would require positioning your hardware with an accuracy of about the radius of a single hydrogen atom.
But that isn't science
There are those who philosophise at great lengths about science as a method. To them science is the tool, and all the lab equipment and so on is a necessary evil that is required to make the tool function. What matters are the great results that make us scratch our heads and rethink our understanding of the world. What gets forgotten in this perspective is that these great new results are usually generated by equipment that was unavailable to the previous generation of scientists.
It is rare that the glory is shared by the instrument makers. So, you can view this column as applause for everyone who spent a few years of their lives putting together a machine for someone else to make all the cool measurements with.
No comments:
Post a Comment
Let us know your Thoughts and ideas!
Your comment will be deleted if you
Spam , Adv. Or use of bad language!
Try not to! And thank for visiting and for the comment
Keep visiting and spread and share our post !!
Sharing is a kind way of caring!! Thanks again!