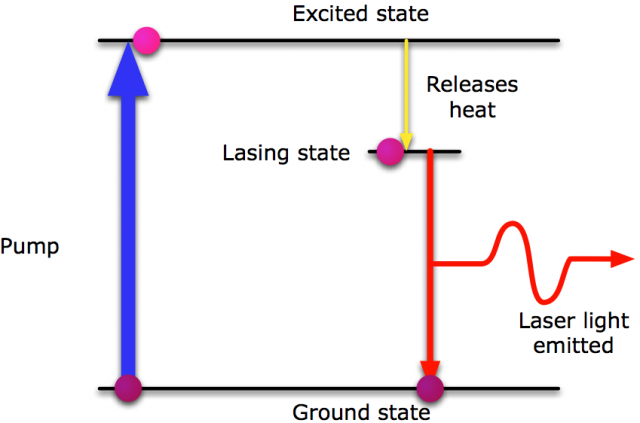
An atom, cycling through the various stages of life in a laser. It starts off all relaxed in the ground state. Then the pump drives it into an excited state. It quickly relaxes into the lasing state, where it waits around for many of its fellow atoms to join it. They then all emit in phase to return to the ground state.
Ever since the first demonstration of the laser, people have wondered if the same thing could be done with sound. Over the last few years, there have been demonstrations that are close but, in my opinion, not quite there. That all changed with a recent publication that is, I think, the first truly convincing demonstration of a phonon laser (a phonon is the sound equivalent of a photon: the smallest increment of energy at a given tone).
Experimentally, what a Japanese team has done is really cool. But unless you happen to be a physicist, to fully appreciate it requires quite a diversion. So, I suggest that you take a moment to read the next section. If you are a physicist, feel free to skip ahead to "The Phonon Laser" section.
What makes a laser a laser?
It is actually harder to define a laser than you might think. For instance, a common description is a light source that has just a single color. Yet there are many lasers that emit a band of colors far broader than many lamps do. So, no, lasers do not necessarily emit a single color.
There are also those who will tell you that lasers emit light in a single direction. But the cheapest lasers of all emit light over an angular range of about 60 degrees. There are plenty of lasers that emit light in every direction, just as you would expect from a lamp.
So, if these two common descriptions of a laser are wrong, what makes a laser? There are, in fact, only two properties that make a laser (though, in special circumstances, you can eliminate one of them). Those are presence of coherence and the detection of a threshold.
Coherence
Imagine that we have a pool of water that is sitting nice and still. We drop a pebble in the water and take pictures of the waves as they expand out. After a couple of pictures, you would be able to predict what the next image would look like. That is because the waves move in a predictable fashion.
Now, imagine it's raining. Each water drop creates its own wave, and each wave is predictable. But because the timing between the waves is random, the overall picture of the ripples in the pond changes unpredictably. A sequence of pictures of the pond would not provide enough information to predict what the pattern of ripples would look like in the following pictures. The wave pattern is incoherent.
We can change the pattern from incoherent to coherent by forcing the rain drops to fall at just the right time. Even though their positions might be random, the timing ensures that the ripples in the pond will build up and form a pattern that is entirely predictable. That is one of the signatures of laser radiation: it forms a predictable pattern in both time and space. This is also the signature of a saser: the sound waves should have certain predictable aspects.
Threshold
The threshold is the amount of power required to make a laser start lasing. As you turn the input power up, the light emitted grows brighter with increasing power at a certain rate until, at the threshold, the brightness increases dramatically. If we go back to coherence for a moment, the threshold is the point at which the light goes from being emitted any old way to only being emitted into a coherent pattern. There are many millions of possible ways to emit light but, at threshold, the light emitters are all forced to make a single choice. Therefore, the efficiency of light production increases by a vast amount.
From a more fundamental perspective, threshold occurs when there are enough emitters ready to emit, and there is enough light to give the emitters a pattern to follow. That is, there needs to be some kind of feedback that reinforces the coherent light pattern. Again, the saser should exhibit a threshold—the amount of sound power emitted into a particular pattern should increase dramatically above a certain input power.
Getting inside the individual emitters
Let's switch things up a little and look at what makes an emitter of light or sound appropriate for a laser or saser. There are only two requirements: the ability to emit light or sound and the ability to store energy. Every atom, molecule, and ion can emit light, so we generally use these to create lasers. Light is emitted when they relax from an excited state to a less excited state.
To make a laser, we want lots of atoms to emit light in phase with each other—or to put it more bluntly, at just the right moment in time. To get that to happen, you require more emitters in the excited state than in the relaxed state. So the problem becomes getting a large population of atoms in an excited state.
The trick is to use an even more excited state (illustrated in the diagram at top). You pump the atoms into this higher excited state, and they can relax to the ground state or into the target excited state. The longer you pump, the more atoms end up in the excited state. Now, when a passing bit of light hits one of the atoms, it triggers it to emit an identical photon, setting off a chain reaction.
OK, now that you have that, lets get to the experimental details.
The phonon laser
The physics of the phonon laser differs from the physics of an optical laser in a very subtle way. In an ordinary optical laser, there are many, many emitters, each of which stores and releases a single photon at a time. In a saser, there is usually a single emitter, one emitter that can store a multitude of phonons. The trick then in a laser is trying to get as many emitters into the right state at the right time; for a saser, you're trying to generate as many phonons as possible in the right state at the right time.
This might sound confusing, but let us consider a simple case where we can magically create a phonon at the right energy in a crystal. When we add our phonon, the crystal begins to vibrate. If we just throw in a second phonon some time later, bad things happen. The two phonons are likely to destroy each other, so that we end up with no motion at all—the phonons both decay to much lower levels and end up heating the crystal up. To add that second phonon to the crystal, we have to do it at just the right time. In other words, the very process of adding phonons to an acoustic resonator has to be coherent.
To get this process to work, the researcher's phonon laser was built around a very special piezoelectric crystal. In a vacuum, this thing will vibrate with incredible precision, and it has a huge number of different frequencies that it can oscillate with, each corresponding to a particular vibrational motion. (Think torsional vibrations and flexing motions, as well as combinations of different motions.) To excite one of these vibrations, you need to put in a phonon of energy with very specific frequency (accurate to one part per million), after which the vibration will persist for around a second. That means that the phonon hangs around in that mode for about a million oscillations before it scatters off something and turns into other, lower frequency phonons.
Normally, this would mean that you drive the mechanical oscillator with a nice electromagnetic wave, which would generate lots of phonons that all eventually decay to lower and lower frequencies, heating up the material that the oscillator is made from. Along the way, though, the phonons can excite other mechanical vibrations. This happens when the phonon splits in two, with each part going into oscillatory modes available to the frequencies of the two new phonons.
So two constraints must be met: when the phonon divides, the energy of the two phonons must be equal to the energy of the phonon that created it; and the mechanical oscillator must have two vibrational modes that match those frequencies. These two conditions are not normally satisfied simultaneously.
Luckily, the researchers had built their oscillator out of a piezoelectric material, which means it can respond to electrical currents. By applying a small voltage, they could strain the oscillator and shift the resonant frequencies. This allowed them to couple three different mechanical modes so that a phonon could decay from a high energy vibrational mode to two lower energy modes of the oscillator.
The researchers showed that when they drove the highest energy vibrational mode, things proceeded as expected, and lower energy phonons appeared. However, once the power exceeded a certain value, the amplitude of oscillations in the two lower energy modes increased very fast. What's more, the oscillations exhibited the sort of coherence—in their case, they measured how predictable the oscillations are—expected for a saser.
Indeed, the oscillation of the lower energy vibrational modes was more coherent than would be expected based on the natural behavior of the oscillator. That is, laser-like behavior forced the oscillator to oscillate more precisely than it would normally.
That was interesting, but not really enough to show a laser-like behavior. See, in that case, they use a nice coherent electrical signal to drive the oscillations, which then divide up into two lower frequency oscillations, both of which proved to be coherent. Nice, but not that unexpected. A laser should also be able to create coherence from an incoherent input of energy.
The researchers demonstrated that as well, though. In that case, they used white noise to drive the high energy vibrational frequency. Once the power was above the threshold, the saser switched on and, even though it was being driven by noise, it oscillated with a very pure signal. And that is pretty convincing evidence.
Will the saser find as widespread application as the laser? I have no clue. The authors suggest a few scientific applications, and I am sure that many other people can think of real-world applications. But, at present, it is simply a great technology demonstration that will be used for studying the quantum properties of macroscopic oscillators.
No comments:
Post a Comment
Let us know your Thoughts and ideas!
Your comment will be deleted if you
Spam , Adv. Or use of bad language!
Try not to! And thank for visiting and for the comment
Keep visiting and spread and share our post !!
Sharing is a kind way of caring!! Thanks again!